To His Coy Mistress (by Andrew Marvell)
Had we but world enough and time,1
This coyness,2 lady,3 were no crime.4
We would sit down, and think which way
To walk, and pass our long love’s day.5
Thou by the Indian Ganges’ side6
Shouldst rubies find7; I by the tide
Of Humber8 would complain. I would
Love you ten years before the flood,9
And you should, if you please, refuse
Till the conversion of the Jews.10
My vegetable11 love should grow
Vaster than empires12 and more slow;13
An hundred years should go to praise
Thine eyes,14 and on thy forehead gaze;15
Two hundred to adore each breast,16
But thirty thousand to the rest;17
An age at least to every part,18
And the last age should show your heart.19
For, lady, you deserve this state,
Nor would I love at lower rate.20
But at my back I always hear
Time’s wingèd chariot21 hurrying near;
And yonder all before us lie
Deserts of vast eternity.22
Thy beauty shall no more be found;
Nor, in thy marble vault,23 shall sound
My echoing song; then worms shall try
That long-preserved virginity,24
And your quaint honour25 turn to dust,
And into ashes26 all my lust;
The grave’s a fine and private place,27
But none, I think, do there embrace.
Now therefore, while the youthful hue28
Sits on thy skin like morning dew,
And while thy willing soul transpires
At every pore with instant fires,29
Now let us sport us while we may,30
And now, like amorous birds of prey,
Rather at once our time devour31
Than languish in his slow-chapped power.32
Let us roll all our strength and all
Our sweetness33 up into one ball,
And tear our pleasures with rough strife34
Through the iron gates of life:35
Thus, though we cannot make our sun
Stand still,36 yet we will make him run.37
Notes:
[1] World enough and time: Marvell’s great poem makes an amatory rather than a cosmological reference to the vastness of space and time, which humanity has gradually come to recognize as the most essential element of the cosmos, implying the general abandonment of our ingrained belief that our world forms the center of the universe. Even among those who recognize these facts, our intuition continues to resist this news, fixing our minds on a motionless Earth around which all cosmic objects wheel in majestic motion.
[2] This coyness: To understand the universe –in general usage, all that exists– requires another suspension of our intuitive understanding. We are, inevitably and by definition, situated within the universe, cannot leave it mentally or otherwise, and therefore cannot hope to acquire an accurate mental image of its full nature.
[3] Lady: Our fundamental comprehension of the overall behavior of the universe arose during the first decades of the 20th century and relied largely upon the unsung contribution of women astronomers at the Harvard College Observatory who “took the measure of the stars,” in Dava Sobel’s phrase, compiling the data that would underpin the first accurate estimates of the distances to faraway nebulae and galaxies.
[4] Think which way to walk: Information has value only to the extent that one knows how to employ it. Led by Henrietta Swan Leavitt, the Harvard women amassed information about a particular type of stars. Known to astronomers as Cepheid variables, after the brightest member of this class, Delta Cephei, these variable stars allowed Edwin Hubble to unlock the secrets of great astronomical distances.
[5] Our long love’s day: Most stars, like our sun, produce an impressively steady output of light, but some vary their brightness in a cyclical, repetitive matter. The Harvard women showed that the length of the interval between peaks in a star’s brightness correlates with the star’s luminosity, or absolute brightness. Comparison of the star’s absolute brightness with its observed brightness then reveals the star’s distance. In 1921, Hubble used his study of Cepheid variables in the Andromeda galaxy to estimate its distance. He demonstrated that, contrary to then-prevailing belief, this galaxy cannot form part of our own Milky Way galaxy, but instead lies far outside it. In 1929, drawing on his continuing use of Cepheid variables to estimate the distances to galaxies, and using other astronomers’ measurements of how rapidly the galaxies are moving away from us, Hubble announced his greatest discovery: the universe is expanding! Hubble found that all but the closest galaxies to our own, which are bound together with our Milky Way by their mutual gravitational attraction, are receding from us, and that the galaxies’ “velocities of recession” increase in proportion to their distances from us. If —and this “if” remains fundamental to our understanding of the universe— we have a representative view of the universe, then other galaxies must see galaxies receding from them, with speeds that increase in proportion their distances from them.
[6] Thou by the Indian Ganges’ side: Hubble’s discovery, combined with the assumption that we have a representative view of the universe, implies that the universe expands everywhere, and that it must have had a beginning in time. The current cosmic expansion might someday reverse into a universal contraction, potentially leading to the rebirth of the universe in a new form. Were this so, modern cosmology would largely conform to the vision of Puranic Hindu cosmology, of a universe in eternal cycles of death and rebirth, with each “day of Brahma,” the longest time in this cosmology, equal to 4.32 billion years.
[7] Shouldst rubies find: To measure velocities, astronomers spread the light from a distant object into its band of colors, called its spectrum, and measure the light’s intensity at each point in the spectrum, denoted by its frequency or wavelength. From long familiarity, astronomers know the pattern of light and dark in a typical galaxy’s spectrum. If they observe this familiar pattern, but find it shifted toward redder or bluer colors, they conclude that the change has arisen from the object’s motion toward or away from the observer. Larger shifts arise from larger velocities, which allows astronomers to measure velocities along the line of sight to an object with high precision. Hubble found that all but the closest galaxies have “velocities of recession,” which produce a “redshift” to longer wavelengths. Among astronomers, the relationship between distant galaxies’ distances and velocities now has the name “Hubble’s law,” with an algebraic expression v = H x d. Here v denotes velocity, d distance, and H, which reveals the cosmic expansion rate, is the “Hubble constant.” Astronomers express this number in units of “kilometers per second [of velocity] per megaparsec [of distance].” One megaparsec (one million parsecs) equals 3.26 million light years, and a light year equals approximately 6 trillion miles or 10 trillion kilometers.
[8] I by the tide of Humber: If objects such as galaxies are receding from one another, they must have been much closer together in the past. Today astronomers use the phrase “big bang” to denote the moment when the present expansion of the universe began, long before any galaxies formed, in a titanic explosion that included all of space along with everything else. (Not easy to imagine!) “Big bang” began as a term of derision from the great British cosmologist Fred Hoyle, born in 1915 in Yorkshire, the largest historical county in England, whose North Sea coast is dominated by the Humber river and its tidal estuary. A brilliant maverick who never received a Ph.D. degree, Hoyle made numerous key contributions to astronomy, including an improved understanding of how stars created the different chemical elements. Although Hoyle accepted the observations that imply an expanding universe, he posited that new matter continuously appears, so the universe forever maintains its “steady-state” appearance, with no origin in a supposed big bang.
[9] Ten years before the flood: In the big-bang model of the cosmos, the earliest moments after the big bang encompassed a tremendous flow of all types of particles, endowed with enormous energies. During the first half hour of the universe’s expansion, countless collisions resulted in the flood of particles that fill the cosmos today. The ongoing expansion of the universe has reduced the average energy per particle, much as the release of steam into a larger volume causes its constituent particles to lose energy and cool down. The vast majority of particles today, so far as we know today, are neutrinos and photons, which spread throughout the universe. Observations of the photon flood from early epochs of the universe, called the “cosmic background radiation,” have confirmed the validity of the big-bang model. (Fred Hoyle never accepted this conclusion, asserting that the photon flood arose from entirely different sources.)
[10] Conversion of the Jews: In 1917, working in the quiet of his office in Berlin, with much of the world consumed by World War I, Albert Einstein wrote a scientific paper containing an equation that applied his new theory of general relativity to the overall behavior of the universe. Einstein’s original form of this equation implied that the universe cannot exist in a static or steady state; instead, the cosmos must always be either expanding or contracting. Neither Hubble, who was then finishing his Ph.D. research and preparing to enlist in the United States Army, nor Hoyle, a two-year-old toddler, had pronounced on this matter, and indeed no astronomers then imagined the universe to be anything but static. Aware of this fact, Einstein “produced” a static universe by inserting an additional term, later called the “cosmological constant,” into his equation. Fifteen years later, with Hubble’s observations confirmed and extended, Einstein told George Gamow that he considered this his “greatest blunder.” Later work, however, has established that cosmologists should include the cosmological constant, though not with the same value that Einstein used, in any equation that describes the expanding universe.
[11] Vegetable: Marvell here references the Earth’s great efflorescence, but the notion applies equally appropriately to the tremendous variety of cosmic objects. Hubble’s observations dealt with what astronomers today call ordinary galaxies, some of whose shapes mimic the spiral structure of our own Milky Way, while others, called elliptical galaxies, have a more diffuse, ellipsoidal distribution of stars. Today astronomers have recognized and classified a much larger cosmic zoo on all size scales, including thousands of extrasolar planets, stars of all ages and colors, stellar explosions, “gamma-ray bursters,” cosmic lasers, masers, quasars, and blazars. Each instrument that opens a new observational window onto the cosmos has revealed new classes of astronomical objects.
[12] Vaster than empires: No one doubts that the immense, nearly incomprehensible vastness of the universe immediately presents a riddle with no known answer: Is the cosmos finite, or is it infinite? Either answer baffles the mind. In a finite universe, space curves back upon itself, so that it resembles the surface of an expanding balloon –except that we must somehow imagine that only this surface, and nothing else, exists. In a finite universe, we take this problem and fling it out to infinity, temporarily a more satisfying outcome, but one that reveals itself as equally difficult to imagine correctly.
[13] And more slow: The problem of conceiving the enormous stretches of time in the universe looms nearly as large as our problem with imagining the extent of space. Perhaps happily, however, the big-bang model of the cosmos allows us, with careful observations and a working theory of the cosmic expansion, to run time’s movie backward in our minds, to conclude that the universe began its expansion close to 13.8 billion years ago. Astronomers are fond of proposing that we imagine reducing these 13.8 billion years to a single year. In that case, the Earth formed in late summer (4.6 billion years ago); life arose around Halloween (3.5 billion years ago); dinosaurs reigned from soon after Christmas until just after noon on New Year’s Eve; hominids appeared a few minutes before midnight, and human history spreads over the final few seconds before the clock chimes.
[14] Praise thine eyes: Through countless millennia, humans could observe the cosmos only with their own eyes. Even on the clearest, darkest nights (far more common before civilization brought forth fire and its illuminatory cousins), our unaided eyes can perceive only a few thousand stars, along with our moon and the five brightest planets. The invention of the telescope four centuries ago marked the first of a stunning series of improvements in our ability to perceive the universe. From Galileo’s primitive instruments –which revealed craters on the moon, Jupiter’s four large satellites, and the nature of dark spots on the solar surface– today’s giant telescopes, both ground-and space-based, can now resolve details thousands of times finer than anything our eyes can see.
[15] On thy forehead gaze: Breaking the bounds of visible light, which occupies only a small portion of the entire electromagnetic spectrum, 20th-century astronomers opened the rest of the spectrum, from gamma-rays, the most energetic photons, through the x-ray and ultraviolet spectral portions, and past visible light to infrared, millimeter, and radio-wave observations. In addition, entirely new means of studying the cosmos have recently become operational, most notably detectors that capture high-energy particles from the sun, exploding supernovae, and other locales of cosmic violence, as well as gravitational radiation, wiggles of space itself produced by the shudder of massive objects.
[16] Two hundred to adore each breast: The amazing zoo of cosmic objects, revealed by studies of the universe in different forms of electromagnetic radiation, can show us only what cosmologists now call “ordinary matter.” This catch-all phrase describes just about everything you may have heard or read about: protons, neutrons, and electrons; photons, muons, neutrinos, and quarks; cosmic rays (misleadingly named streams of some of the particle types just listed); neutron stars and pulsars; quasars and blazars; and other objects that appear in the news from time to time. Once upon a time –during the youth of now-aged astronomers– this totality was thought to be all that the universe contains. Today we have found this description significantly lacking.
[17] Thirty thousand to the rest: Cosmologists now conclude that all types of ordinary matter, measured in energy terms by applying Einstein’s famous equation E = mc2, amount to only about 5 percent of the total energy budget of the universe. The bulk of that budget consists of “dark matter” and “dark energy.” These two phrases denote entirely different phenomena with misleadingly similar names, though neither can be perceived through the use of any type of electromagnetic radiation. “Dark matter” describes matter that by definition cannot be detected in this way; instead, astronomers find it by observing dark matter’s gravitational effects on visible matter, and know nothing about the actual nature of dark matter beyond these effects. For example, dark matter in the Milky Way and other giant galaxies affects the orbital speeds of stars that circle the galaxies’ centers. In contrast, “dark energy” refers to a mysterious energy, unlike any familiar sort, that lurks in every cubic centimeter of the universe and is born in increasing amounts as the universe expands to produce new space. Among the universe’s energy budget, dark matter furnishes 27 percent of the total, while dark energy, the dominant contributor, provides 68 percent.
[18] An age at least to every part: The big bang produced the different particles that now fill the universe, with some of them –photons and neutrinos– diffusing through all of space, while others have created local clumps such as planets and stars. Dark matter’s “clumpiness” remains largely a mystery, though it has become fairly clear that its distribution roughly mimics that of the matter in galaxies and galaxy clusters. In contrast, dark energy fills all of space,with the mind-bending quality that new dark energy continuously appears as the universe expands. The 5/27/68 distribution of ordinary matter/dark matter/dark energy, therefore refers only to the present time, not to the past or the future. Instead, dark energy’s contribution has grown from essentially zero into its dominant position today, and this domination will only increase as the universe continues its expansion.
[19] The last age should show thy heart: Dark energy behaves in a mysterious but straightforward manner: It makes space expand. An increase in dark energy therefore implies a greater expansionist tendency: The universe now expands more rapidly than it did in bygone eras, and will expand ever more rapidly as the future arrives. This startling result, verified by observations that look far back in time by studying far-distant supernova explosions, shook the cosmological world during the mid-1990s. Today cosmologists accept this fact, a natural accompaniment to the existence of dark energy in an expanding universe. The last age of the universe will therefore be one of near-complete cosmic darkness and emptiness (so far as ordinary and dark matter are concerned). With stars and other objects burnt out, and all of them removed from one another unless bound by gravitational forces in cosmological proximity, the universe will consist almost entirely of dark and empty space –except that space will contain ever-growing amounts of the dark energy that will have driven the cosmos to this sad (at least for astronomers) state.
[20] Love at lower rate: During the past few years, a significant controversy –called a “crisis” by some cosmologists– has arisen over a fundamental descriptor of the cosmos: Just how rapidly is the universe expanding? Astronomers now have several different methods for measuring the cosmic expansion rate, which now disagree. Cosmologists throughout the world are arguing about the significance and explanation of this divergence.
[21] Time’s wingèd chariot: Hubble’s method for estimating distances relied on using Cepheid variable stars as “standard candles,” objects that all have the same luminosity or intrinsic brightness, so that a comparison of their observed brightnesses reveals which of them are more distant, and by how many times. This method works well for comparatively nearby galaxies, but fails for more distant ones, because their Cepheid variable stars cannot be seen and measured as individuals. During the 1990s, astronomers found a more luminous species of standard candle in a particular type of supernova explosions, the enormous final gasps of highly evolved stars. They realized that this type, recognizableby the characteristics its spectrum of light, that all reach the same maximum luminosity. The most recent and detailed studies of cosmic expansion using this supernova method, reported by a group of astronomers led by Adam Riess, who won a Nobel Prize in physics for his role in the use of these supernovae in the discovery of dark energy, sets the Hubble constant at 74.4 ± 1.4 kilometers per second per megaparsec. The number following the “±” denotes the estimated error in deriving this result, statistically named “one sigma of standard deviation.” Under normal statistical analysis of repeated events, an error of one sigma in measurement occurs quite often, but one twice as large (“two sigma”) occurs only once in twenty times among similar sets of data. (When political polls cite their possible errors, they use plus or minus two-sigma.) The supernova result for the Hubble constant clashes with one described below, not by one or two sigma but by four or more.
[22] Deserts of vast eternity: Cosmologists now possess a completely different approach to determining the universe’s expansion rate, one that depends on detailed measurements of the cosmic background radiation. This radiation, created during the first moments of the big bang, passed its first few hundred thousand years in an intimate relationship with the matter in the universe. That epoch was characterized by countless collisions among the photons that form the background radiation and all the particles of different types that provide the mass of ordinary matter. Throughout those years, no atoms could form without being immediately destroyed by impacts from high-energy photons. Had this situation continued indefinitely, the universe would have remained without individual objects such as stars and galaxies—an eternity of formlessness, maintained by the flood of photons created in the fury immediately after the big bang.
[23] Marble vault: The continuing expansion of the universe steadily robbed this flood of photons of their energy. Eventually each photon had too little energy to break an atom apart. As a result, from then on, photons have basically ceased to interact significantly with other forms of matter. Since that “time of decoupling,” 380,000 years after the big bang, the photons and matter have gone their separate ways, only rarely interacting. The cosmic background radiation of photons continues to fill the universe, with the photons continuing slowly to lose energy. But the radiation provides a hugely important cosmic relic, a record of the cosmic situation at the time of decoupling. In particular, the radiation has some regions slightly richer in photons than average, and others slightly poorer –only by a few parts in a hundred thousand, but now susceptible to detection and detailed study. The variations in photon intensity reveal which regions of space were slightly denser than average at the time of decoupling. These are the regions that became the largest assemblages of matter– the giant clusters of galaxies that we see today. Cosmologists can now apply their analysis of the universe’s development to unlock the marble vault of cosmic structure formation, tracing its overall development from the time of decoupling to the present day.
[24] Long-preserved virginity: Cosmologists can study the history of how tiny deviations from a perfectly smooth distribution of matter grew into clumps of matter on the largest distance scales because they now understand the physics of the universe in its earliest stages as well as in later times. For example, they can calculate in any epoch the speed of sound, the limiting speed at which particles with mass can move in most circumstances. In particular, they can determine the speed of sound at the time of decoupling. At that time, the largest “structures” –the regions slightly denser than average– had a diameter equal to the sound speed times 380,000 years, which turns out to be approximately 490 million light years. Cosmologists can apply this fact to their observations of both the cosmic background radiation and the current distribution of matter on the largest distance scales in order to determine the cosmic expansion rate throughout the universe’s post-decoupling history. In particular, they can use this analysis to calculate the current value of the Hubble constant, which turns out to be 67.4 ± 1.2 kilometers per second per megaparsec.
[25] Quaint honour: The Hubble-constant result from the cosmic background radiation differs from the one obtained from supernova observations by more than four sigma. This divergence must arise either from significant errors in obtaining and analyzing the observational data, or from another explanation, which might involve new laws of physics. The disagreement has provoked impressive controversies among astronomers and cosmologists, who often see their honor at stake when accused of having made errors in their observations or data analysis. (Today’s readers of Marvell’s poem are likely to overlook the earthy meaning of his phrase “quaint honour,” which appears, with more direct implication, in “The Miller’s Tale” by Geoffrey Chaucer.) Before discussing this controversy, which some cosmologists refer to as a “tension” and others as a “crisis,” we should note that several additional methods now exist to determine the Hubble constant.
[26] Ashes: Another method, conceptually related to the observations of distant supernovae, finds “standard candles” not in individual objects but rather in the evolution of a large cluster of stars, within which, astronomers reasonably assume, the stars were all born at nearly the same time. Each star’s subsequent evolution depends on its mass, with more massive stars aging more rapidly. As the stars age, some of them in later life swell their outer layers while contracting their cores to become “red giants” of great luminosity. The most luminous of all the red giants tend to attain the same maximum luminosity in any cluster. By studying the colors and brightnesses of the cluster’s stars, astronomers can determine the red giants that have reached this maximum, called, in a lovely phrase that astronomy can produce, the “tip of the red giant branch.” This tip allows the clusters to serve as standard candles. A recent study using this method has found a value of the Hubble constant equal to 69.8 ± 1.7 kilometers per second per megaparsec.
[27] The grave’s a fine and private place: A fourth, entirely independent, method for determining the Hubble constant relies on “gravity waves.” In 1916, Albert Einstein predicted that ripples in space, more precisely known as “gravitational radiation,” would arise whenever massive objects move rapidly in close proximity. Ninety-nine years later, amazingly sensitive detectors in Louisiana and Washington State made the first direct detection of gravitational radiation; two years after that, these two instruments, plus another in Italy, detected gravity waves from the sudden merger of two collapsed neutron stars. In this case, astronomers for the first time observed a gravitational-radiation event in ordinary light that allowed them to measure the objects’ recession velocity. Because they understand how these mergers proceed, the gravitational radiation’s time variation provides a “standard siren” that reveals the intrinsic “luminosity” strength of the gravitational radiation produced by this neutron-star merger. Comparison with the observed strength of the radiation yields the distance to the event.
[28] Youthful hue: Although the gravitational-radiation approach to determining the Hubble constant remains in infancy, the analysis of observations of the neutron-star merger observed in 2017 yielded a value for the Hubble constant of 74 (plus 16 or minus 8) kilometers per second per megaparsec. This result, with its somewhat confusing statement of errors –larger errors than those for the results described above– deserves respect for the fact that a single gravitational-radiation observation has already provided important information. During the next decade or two, as astronomers should observe many more such events, they will furnish another method, potentially equally accurate, for determining the constant that has provoked so much controversy.
[29] Instant fires: What are we to make of the results cited above, with the striking divergence of results from the results from the studies that rely on observations of supernovae, the cosmic background radiation, and the tip of the red-giant branch in distant star clusters? When asked for their reaction, theoretically-oriented cosmologists tend to sigh as they emphasize the immense difficulties that these observations and their data reduction inevitably face as they stand at the edge of our current capabilities. History has shown that many a tremendous “conflict” has evaporated as better data has emerged. In sometimes angry contrast, observationally oriented cosmologists, who have often worked for years to obtain these results, rebut this easy-going approach and insist that they imply real and significant problems before we can conclude that we possess a full understanding of the behavior of the universe since its earliest moments.
[30] While we may: Everything so far discussed in this essay has assumed the validity of what cosmologists now call their “standard model” of the universe, summarized in an acronymic way as the “LCDM model,” in which L (Greek lambda) stands for the cosmological constant, and CDM for “cold dark matter,” meaning dark matter consisting of particles that move at speeds far less than the speed of light. The LCDM model has worked so well, up to the present time, at describing the observed universe that almost all astronomers and cosmologists hesitate mightily about modifying, let alone abandoning, its comparative simplicity.
[31] Our time devour: The fertile minds of theoretically oriented cosmologists have suggested various modifications to the standard LCDM model. Some of these may be tested in the near future, while others must await far better improved observational capabilities before we can assess their relationship to reality. The best-known (at least among cosmologists) of these suggestions include changes to Einstein’s theory of gravitation, often referred to as MOND –“modified Newtonian dynamics”– even though Einstein’s gravitational theory already modified Newton’s (although these modifications do not affect the areas probed by the suggested changes). Other cosmologists suggest that currently unknown species of particles, or hitherto unsuspected interactions between dark energy and dark matter, could affect the standard model in ways that might explain the current divergence among observational determinations of the cosmic expansion rate.
[32] Slow-chapped power: The history of science shows that time works wonders when humans attempt to understand and to explain the universe. The 20th century saw the emergence, among other startling events, of the quantum theory, relativity, the harnessing of nuclear fission and the understanding of nuclear fusion. The first fifth of the 21st century has produced nothing so massive in impact as these, but the century remains fairly young. Our grandchildren, who should live well into the 22nd century, have a good chance to learn what has made the cosmos behave as we see it.
[33] All our sweetness: A scientific truism, nowhere more evident than in astronomy, states that whenever we invent or introduce improved methods of observation, unexpected discoveries follow. Building on the past few decades, which have given us not only dark energy and gravitational radiation but also thousands of exoplanets, images of a black hole, and a host of new results about objects in the solar system, we may confidently await new insights that will flow from the operation of the great new instruments that astronomers will soon deploy in their observations of the cosmos.
[34] Rough strife: The great challenge that humanity now faces resides in the question of how we can reconcile the result of our slow evolution, which made us fit to live in small groups, with the world that we have now created, one of rapidly growing human population and the changes in terrestrial climate and the worldwide ecological system that we have produced.
[35] Iron gates of life: The long sweep of cosmic history has seen the birth of the sun and its planets, 4.6 billion years ago; the appearance of life on Earth a few hundred million years later; and the long sweep of biological evolution on our planet that has given us all that we have –our bodies, our nourishment, our understanding, and the entire ecological web that sustains us, and whose diminution we risk at great peril.
[36] We cannot make our sun stand still: Writing in the mid-17th century, Marvell knew well that Copernicus had dethroned the Earth, and uses this metaphor to reflect what we see rather than what actually occurs in the heavens.
[37] Make him run: Our cosmically brief lives give each of us a chance to connect with the universe, drawing on the knowledge that we inherit from human generations before us and from the work that goes on around us. May it continue!
Figure 1 – In this Hubble Space Telescope photograph of the galaxy cluster designated as SDSS J0146-0929, each extended point of light is a galaxy containing many billion stars. At the cluster’s center, we see that the gravitational force from two large galaxies has bent the light from much more distant galaxies, producing arcs that form part of what astronomers call an “Einstein ring” in honor of the scientist who first predicted that gravity bends light.

https://www.nasa.gov/image-feature/goddard/2018/hubble-finds-an-einstein-ring
Figure 2 – This Hubble Space Telescope photograph captures the remnant of a supernova, seen about a thousand years after its explosion. Called the Crab Nebula, this expanding web of gas and dust conceals at its center a collapsed neutron star, the remnant of the once-mighty star whose final outburst expelled the material visible around it.
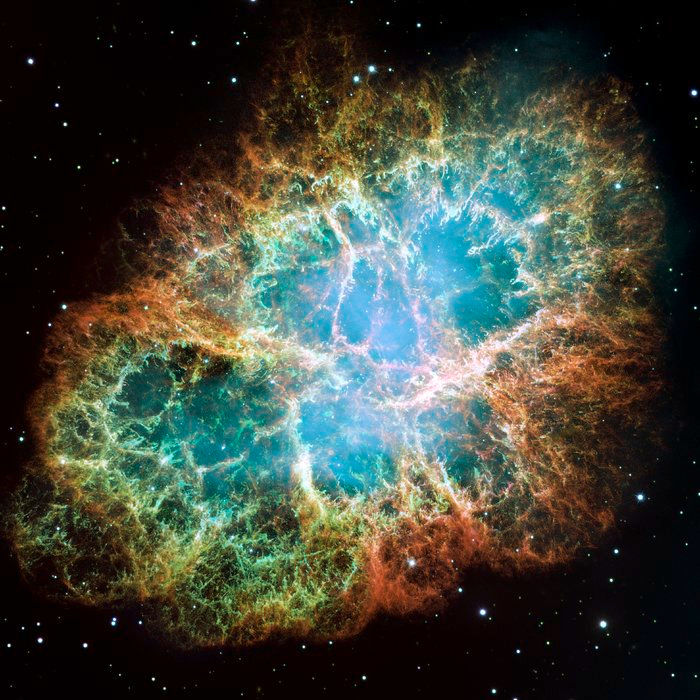
https://www.spacetelescope.org/images/heic0515a/
Donald Goldsmith, an astrophysicist and attorney, has received a Ph.D. in astronomy and a J.D. in law, both from the University of California at Berkeley. After half a decade as a research astronomer and faculty member, Dr. Goldsmith has devoted himself primarily to the popularisation of science. He is the author or co-author of 15 books, including The Astronomers, Exoplanets, Origins (with Neil deGrasse Tyson), and the forthcoming The End of Astronauts (with Martin Rees), and has served as a consultant or co-writer for television documentary programmes and series such as Carl Sagan's "Cosmos," "The Astronomers," Neil Tyson's "Cosmos," and "Is Anybody Out There? with Lily Tomlin."
1